Crop management and its impact on soil health and carbon
| Date: 19 Jun 2008
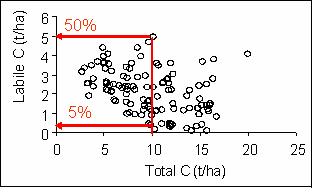
Frances Hoyle, L&WA, DAFWA
Daniel Murphy, UWA
KEY MESSAGES
• Identify the key chemical, physical and biological constraints to reaching rainfall limited yield potential – address key constraints first
• Retain organic matter to enhance nutrient cycling, biological activity and soil stability
• Natural levels of soil organic carbon vary with rainfall
• The ability of a soil to store carbon depends on climate, soil texture and land use
• Moist, hot and well-aerated conditions favour microbial activity and the rapid decay of organic additions
• Soil organic matter is not all the same – it is composed of readily available (labile) pools through to very stable pools.
• It is often difficult to measure changes in the total soil organic matter pool, whilst labile pools of carbon change relatively rapidly.
Background
Agricultural management practices seek to optimise plant and animal productivity within the constraints of climate, and the capacity of the soil (physical, chemical and biological attributes) to support plant growth. Whilst we understand many of the physical and chemical conditions required, we have a much poorer understanding of the control that biological factors have on plant growth. The objective of this paper is to examine the relative contribution of soil biological attributes, including organic carbon to crop production using Western Australian (WA) farming systems as an example.
Identifying Soil Constraints to Crop Production
Environmental indicators are measures of physical, chemical, biological or socio-economic characteristics which reflect changes in processes, properties or function - with different attributes required for different land uses. Since it is not practical to measure all soil characteristics, a minimum set of key attributes should be defined that are designed to demonstrate whether soil resources are being depleted, maintained or built up. These are likely to include whether a soil is losing or gaining organic matter, shifts in soil pH and changes in soil nutrient supply. Once soil constraints are identified their economic importance can be assessed (i.e. cost and practicality of removing the constraint versus potential yield benefit) prior to implementing changes in agronomic practice. Often, paddocks not achieving their yield potential (defined by available water) will demonstrate multiple constraints – the most limiting factor should be the first one addressed.
Soil organic carbon
Soil organic carbon (SOC) is a measure of the organic C content of soils – reflective of organic matter inputs from both living (active roots; soil fauna such as earthworms, termites, mites, fungi, bacteria, nematodes) and non-living (root exudates, decaying plant and animal material, humus and charcoal) sources. Changes in SOC reflect the balance between inputs and outputs (including CO2 from microbial decomposition and any direct losses from erosion).
Sustainable management of soil, in particular soil organic matter (SOM), is essential for the continued viability of the WA agricultural sector. SOM plays a key role in C, N, S and P cycling and also acts to improve soil structure. Agricultural practices and plant inputs influence both the quantity and quality of SOM, which directly impacts on soil productivity, soil resilience and soil sustainability. For example, soil texture influences the capacity of a soil to retain C. Clay platelets coat organic matter to form stable aggregates, physically protecting organic material from microbial decomposition – thus heavier soils have a greater capacity to stabilise organic carbon. In comparison, rapid turnover of organic material occurs in soils with little or no clay content and explains why increasing organic carbon in coarse textured sandy soil is comparatively difficult. Increased soil disturbance also contributes to more rapid break down of soil aggregates and exposes residues and other soil organic matter to microbial decomposition, accelerating the rate of soil C decline.
The soil is composed of approximately 90-98% minerals and only 2-10% organic matter. Of the SOM present in soil, approximately 15% of this is ‘living’ (made up of roots, fauna and micro-organisms). Micro organisms are the predominant component of this ‘living’ pool of organic matter and as they turn over rapidly are considered essential for organic matter decomposition and nutrient cycling, degradation of chemicals and soil stabilisation.
Soil organic carbon fractions
In soil, organic carbon exists in fractions or ‘pools’ that vary in size, chemistry and decomposability. Some of these are readily available and turnover rapidly (i.e. are more labile), whilst others break down more slowly and contribute to the more stable organic carbon pools.
In WA, although significant amounts of SOM are present in agricultural soils, some of the carbon is relatively inert and is associated with highly weathered soils and historical burning. The labile pool of carbon is primarily influenced by ‘new’ organic matter (originating from plants and/or animals) and provides an energy source for biological activity, and is important in both nutrient cycling and soil structure. The higher the labile component of the total SOM pool, the higher the biological fertility status of the soil. In Figure 1, a range of soils have a measurable total SOM pool of 10 t/ha (equivalent to 1.0% SOC at a bulk density of 1.0). However, the soil with 50% of its total soil organic matter present as a labile pool, suggests a more biologically active soil with greater potential for nutrient turnover than the soil with 5% labile carbon (Figure 1).
Figure 1 The quantity of labile carbon (t/ha) as a proportion of the total soil organic matter content (t/ha) in WA arable soils.
Nutrient cycling
Organic residues are often discussed in terms of their quality (defined by the carbon to nitrogen or ‘C/N ratio’ of organic materials) since this influences N supply and the potential storage of C in soil.
The extent to which nutrients are released depends on the decomposition rate and C/N ratio of organic residues, and the interaction with decomposer communities and environmental conditions.
The capacity of micro-organisms to release plant-available N is influenced by the quality of organic matter inputs (Figure 2), with net release of N occurring where N supply is in excess of microbial demands. Organic matter with a high C/N ratio (i.e. wheat), breaks down more slowly than residue with a low C/N ratio (i.e. legumes) and is more likely to contribute to increasing soil carbon. Inputs of more recalcitrant residues (e.g. wheat stubble) can result in net immobilisation of N from the soil, making it unavailable for plant uptake. Thus strategic fertiliser inputs are required to optimise N supply.
Figure 2 The relationship between quality of labile carbon (C/N ratio) and soil nitrogen supply (kg N/ha) in WA arable soils.
Effect of soil and crop management on carbon and nitrogen cycling
Grain production in WA primarily occurs in areas where average annual rainfall is between 325 and 750 mm, most of which falls during the winter growing season. Soils in this region tend to be highly weathered with low surface clay and soil organic matter (SOM) content. Summer is typified by hot dry conditions with infrequent storm events, largely restricting production to an annual winter cropping phase. Rainfall therefore constitutes the primary constraint (environmental) to OM production and SOM accumulation. Although low biomass and the lack of new plant residues to soil (as a carbon food source) present a significant challenge to the build up of SOM and biological function compared to temperate environments, this does not mean that soil biology is not important. Indeed, the WA farming system is reliant on a cyclic pattern of biological activity which ‘explodes into action’ with rainfall.
Soil N released from crop residues and SOM results primarily from the activity of micro organisms and can supply up to 80% of the crop N requirements - therefore any change in their mass, or activity can result in changes to biological soil N supply. Plant residues are a primary form of organic matter utilised by micro organisms for microbial growth and activity. Thus increasing organic inputs and the retaining crop residues provides a practical means of increasing the size of the microbial population. In a low rainfall (< 325 mm) environment on a red-brown earth, stubble retention resulted in up to 45% greater microbial biomass in the surface layer (0-5 cm) of the soil, and doubled the biological activity compared to burnt areas. In this trial, the microbial biomass (measured to 30 cm depth) contained the equivalent of 192 kg/ha urea (stubble retained) and 146 kg/ha urea (stubble burnt) - a significant source of potentially plant available N. These results illustrate that regular inputs of organic residues promote both a larger but also more active microbial community.
Best practice principles for increasing soil organic carbon
When considering management of SOM it is important to define the targeted outcome, as this will influence the relevance of specific aspects of crop or soil management (Figure 3). A prevalent question to ask is, if there is more C in my soil does it have a benefit and what is that benefit? For example, adding charcoal to soil in sufficient quantities would make both the C content greater (if added at a high enough rate) and the colour darker, but it would not increase the soil’s fertility. Alternatively, fresh inputs of crop residues are relatively labile and enhance soil fertility, but are unlikely to have a significant role in sequestering carbon. Therefore, in managing soils for SOC it is important to determine purpose.
Figure 3 Management practices to increase soil organic carbon and improve soil function.
Adapted from Leslie Cooperband (2002) Building Soil Organic Matter with Organic Amendments, University of Wisconsin-Madison; Centre for Integrated Agricultural Systems.
Soil OM is in a constant state of turnover, where it is decomposed and replaced by new organic material. To build up SOM to its potential (determined by soil type and climate) under a managed cropping or grazing system you need to add organic material, monitor SOM levels and reduce SOM losses.
• Add organic material
- Grow high yield, high biomass crops and maximise crop frequency
- If available locally, apply manure/compost
- Include pasture rotations and perennial plants where possible and use pastures in the inter-rows for tree crops and vines
- Green manures (options for no tillage systems available) and cover crops
• Reduce losses
- Eliminate unnecessary cultivation (introduce zero or minimum tillage)
- Retain crop residues
- Maintain and conserve ground cover - To maximise wind erosion control, stubble should cover a minimum of 50% of the soil surface, preferably be standing (anchored by roots) and be a minimum height of 10 cm
• Monitor to assess whether management change is depleting or restoring the natural soil resource
Although factors related to the quality, quantity and placement of organic matter affects its rate of decomposition, in total, between 40 and 75% of organic carbon in crop residues and animal by-products added to soil may be respired as CO2 by micro-organisms and lost from the soil. This is influenced by soil texture and climate.
ACKNOWLEDGEMENTS
This research was funded in part by Land & Water Australia, The Grains Research and Development Corporation with support from the Department of Agriculture and Food Western Australia and The University of Western Australia.
Contact: Frances Hoyle
Ph: 02 6263 6047
Email: frances.hoyle@lwa.gov.au
Contact: Daniel Murphy
Email: dmurphy@cyllene.uwa.edu.au
L&WA / GRDC Project UWA54; GRDC Project UWA395
Was this page helpful?
YOUR FEEDBACK